Lithium-ion battery energy density is at the heart of modern technological advancements. It determines how much power can be packed into a given space or weight, making it crucial for everything from portable electronics to electric vehicles. In this blog, we explore the science behind energy density, its impact on various industries, and the innovations that are pushing the boundaries of what batteries can achieve. Whether you’re a tech enthusiast, an engineer, or simply curious about the future of energy storage, this deep dive will shed light on why energy density matters and how it is shaping our world.
1. What Is Lithium-Ion Battery Energy Density?
Lithium-ion battery energy density refers to the amount of energy a battery can store per unit volume (volumetric energy density in Wh/L) or per unit weight (gravimetric energy density in Wh/kg). Simply put, it determines how much power a battery can hold relative to its size or mass.
Why Does Energy Density Matter?
- Higher energy density means longer runtime for devices (e.g., smartphones, EVs).
- Lighter and more compact batteries improve portability (e.g., drones, wearables).
- Critical for industries like electric vehicles (EVs) and renewable energy storage.
How Is It Measured?
Energy density is calculated by dividing the battery’s total energy capacity (Wh) by its weight (kg) or volume (L). For example:
- A 300 Wh/kg battery can deliver 300 watts of power for one hour per kilogram.
2. How Energy Density Relates to Battery Structure
The energy density of a lithium-ion battery depends on its internal components:
Key Structural Factors:
-
Electrode Materials
- Anode: Graphite (common) vs. silicon (higher capacity but less stable).
- Cathode: NMC (Nickel-Manganese-Cobalt), LFP (Lithium Iron Phosphate), etc. NMC offers higher energy density than LFP.
-
Electrolyte
- Liquid electrolytes (standard) vs. solid-state (emerging, potentially 2x energy density).
-
Cell Design
- Pouch cells (lightweight, medium density) vs. cylindrical cells (e.g., Tesla’s 4680, optimized for density).
Why Do These Matter?
- Cathode chemistry alone can increase energy density by 20–30%.
- Silicon anodes (theoretical capacity: 10x graphite) are a major research focus.
3. Battery Production Workflow & Its Impact on Energy Density
Step-by-Step Production Process:
-
Electrode Manufacturing
- Coating anode/cathode materials on foil (precision affects consistency).
- Critical: Uneven coatings reduce energy density.
-
Cell Assembly
- Stacking electrodes and separators (dry vs. wet methods impact density).
- Laser cutting minimizes material waste.
-
Electrolyte Filling
- Insufficient electrolyte lowers ion conductivity; excess increases weight.
-
Formation & Aging
- Slow charging/discharging cycles stabilize capacity (prevents early degradation).
Key Improvements for Higher Density:
- Dry electrode tech (Tesla’s approach: eliminates solvents, boosts density by 5–10%).
- Precision calendaring (thinner, denser electrodes).
4. How the Working Principle Affects Energy Density
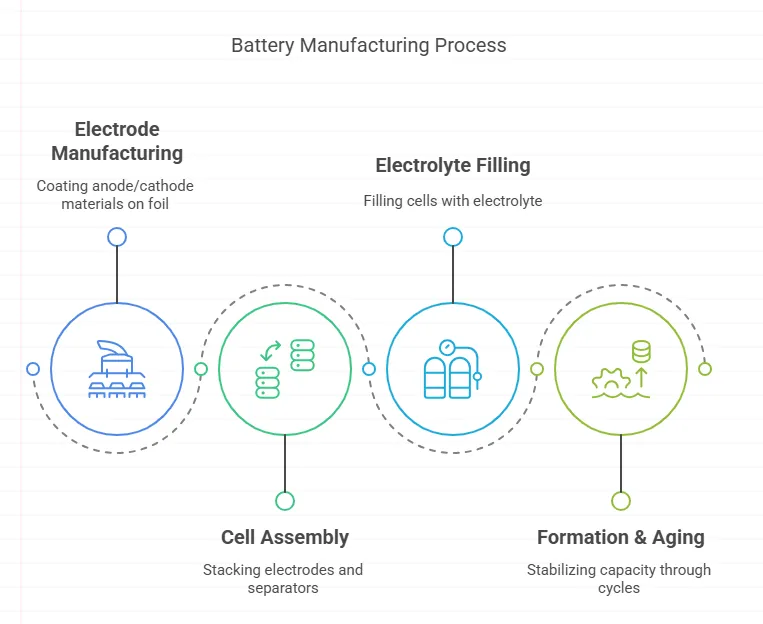
Core Mechanism:
Lithium ions move from the cathode to the anode during charging (reverse during discharge). Energy density depends on:
-
Ion Mobility
- Faster movement = higher power output (e.g., high-nickel cathodes improve mobility).
-
Material Stability
- Volatile chemistries (e.g., nickel-rich cathodes) may degrade faster, reducing usable density over time.
Why Do Some Batteries Degrade Faster?
- Side reactions (e.g., lithium plating) consume active materials.
- Poor thermal management accelerates capacity loss.
Innovations to Watch:
- Solid-state batteries (no liquid electrolyte, safer, 2–3x theoretical density).
- Silicon nanowire anodes (experimental: 3,750 mAh/g vs. graphite’s 372 mAh/g).
5. What Are the Key Applications of High-Energy-Density Lithium-Ion Batteries?
High-energy-density lithium-ion batteries power modern technology by storing more energy in smaller, lighter packages. Here’s where they make the biggest impact:
Why High Energy Density Matters in These Applications:
-
Electric Vehicles (EVs):
- Longer driving ranges (e.g., Tesla’s 4680 cells offer ~300 Wh/kg).
- Reduced weight improves efficiency.
-
Consumer Electronics:
- Slimmer smartphones (e.g., Apple iPhone) and longer-lasting laptops.
- Enables compact wearables (e.g., wireless earbuds).
-
Aerospace & Drones:
- Extended flight times for UAVs (e.g., DJI drones).
- Lightweight power for satellites and electric aircraft.
-
Grid Storage:
- Higher capacity for renewable energy storage (e.g., Tesla Megapack).
Emerging Uses:
- Medical implants (e.g., pacemakers with longer lifespans).
- Military equipment (high-capacity portable power).
6. What Is the Optimal Working Environment for High-Energy-Density Batteries?
Lithium-ion batteries perform best under specific conditions. Deviations can reduce efficiency or safety.
Ideal Conditions:
-
Temperature Range:
- Charging: 0°C to 45°C (32°F to 113°F).
- Discharging: -20°C to 60°C (-4°F to 140°F).
- Why? Extreme cold slows ion movement; heat accelerates degradation.
-
Humidity:
- Low-moisture environments prevent corrosion.
-
Voltage Limits:
- Overcharging (>4.2V/cell) causes lithium plating; deep discharge (<2.5V) damages electrodes.
Step-by-Step Guide to Maximizing Battery Life:
- Avoid Extreme Temperatures – Store at 20°C (68°F) when possible.
- Use Partial Charging (20%-80%) – Reduces stress on electrodes.
- Prevent Physical Damage – Punctures can lead to thermal runaway.
7. How Is Energy Density Tested and Quality Controlled?
Manufacturers use rigorous testing to ensure batteries meet energy density claims.
Key Testing Methods:
-
Capacity Measurement (Wh/kg or Wh/L)
- Discharge battery at controlled rates to measure total energy output.
-
Cycle Life Testing
- Repeated charging/discharging to simulate aging (e.g., 500+ cycles).
-
Thermal Stability Tests
- Expose cells to high temps to check for leaks or swelling.
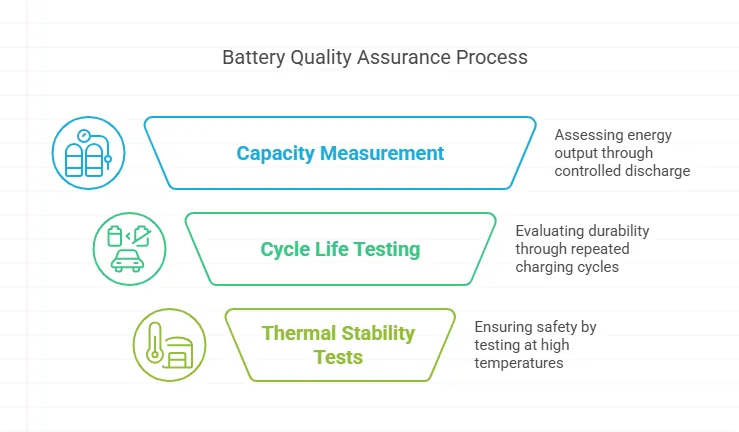
Quality Control Steps:
- Electrode Coating Uniformity – X-ray scans detect inconsistencies.
- Impurity Screening – Trace metals degrade performance.
- Final Performance Validation – Batch sampling ensures consistency.
Why Testing Matters:
- A 5% variation in electrode thickness can reduce energy density by 10%.
- Tesla’s in-house labs test cells under real-world conditions.
8. What Are the Key Usage Precautions for High-Energy-Density Batteries?
Higher energy density increases power but also risks if mishandled.
Critical Safety Rules:
✅ Do:
- Use manufacturer-approved chargers (prevents overvoltage).
- Store at ~50% charge if unused for months.
- Keep batteries dry and clean.
❌ Don’t:
- Expose to temperatures >60°C (140°F) – risk of thermal runaway.
- Pierce or crush cells – electrolyte is flammable.
- Charge damaged/swollen batteries.
Step-by-Step Emergency Response for Damaged Batteries:
- Isolate the Battery – Move to a non-flammable surface.
- Avoid Water – Use a Class D fire extinguisher for lithium fires.
- Contact Professionals – Do not attempt to repair leaking cells.
Industry Standards:
- UN/DOT 38.3 certification for shipping safety.
- UL 2054 for consumer electronics compliance.
This blog provides an in-depth look at lithium-ion battery energy density, a critical factor in modern energy storage solutions. We define energy density and explain its significance, including how it affects device runtime, portability, and overall performance. The article examines the key structural components of lithium-ion batteries, such as electrode materials and electrolytes, and how they influence energy density. We also explore the production workflow, highlighting steps like electrode manufacturing and cell assembly that can enhance or hinder density. Additionally, we discuss the working principles that affect energy density, including ion mobility and material stability. The blog highlights the key applications of high-energy-density batteries, from electric vehicles and consumer electronics to aerospace and renewable energy storage. Finally, we cover the optimal working conditions for these batteries and the essential testing and safety precautions required to ensure their reliability and longevity.