Lithium-ion battery life is a critical factor in the performance and longevity of modern devices, from smartphones to electric vehicles. How long your battery lasts can depend on a variety of factors, including how it’s measured, how it’s designed, and how you use it. In this article, we explore the key metrics of battery life, such as cycle life and capacity retention, and uncover how cell design, charging habits, and environmental conditions impact longevity. Whether you’re looking to extend the life of your personal device or manage large-scale battery systems, this guide provides essential insights and practical tips.
How is Lithium-Ion Battery Life Measured? (Cycles, Capacity Retention)
What Determines Lithium-Ion Battery Life?
Battery lifespan is measured via cycle life and capacity retention:
- Cycle Life: The number of complete charge-discharge cycles before capacity drops to 80% of its original value.
- Capacity Retention: The remaining energy storage capability after aging (e.g., 90% after 500 cycles).
Why Do These Metrics Matter?
Manufacturers use them to predict usability for EVs, phones, or grid storage. A battery with 1,000 cycles (at 80% retention) may last ~3–5 years in daily use.
How Testing Works (Step-by-Step)
- Charge/Discharge Cycling: Batteries are repeatedly charged to 100% and drained to 0% under controlled conditions.
- Capacity Tracking: Tools measure voltage decay and energy loss per cycle.
- Environmental Simulation: Tests include high-temperature aging to accelerate degradation.
Cell Design Choices and Their Effect on Longevity
Why Cell Design Impacts Battery Life
Materials and structure dictate stress resistance. Key factors:
- Anode Material: Silicon anodes store more energy but crack faster than graphite.
- Cathode Chemistry: NMC (Nickel-Manganese-Cobalt) offers longevity; LFP (Lithium Iron Phosphate) excels in cycle life.
- Electrolyte Additives: Stabilize electrodes to reduce side reactions.
How Design Extends Lifespan
- Thicker Electrodes: Higher energy density but slower ion flow (trade-off).
- Stacking vs. Winding: Pouch cells (stacked) cool better than cylindrical (wound), reducing heat degradation.
Charging/Discharging: How It Accelerates Battery Aging
What Happens During Cycling?
- Li-Ion Shuttling: Ions move between anode/cathode, causing physical stress.
- Heat Generation: High currents or extreme temperatures accelerate chemical breakdown.
Why Charging Habits Matter
- Voltage Stress: Keeping a battery at 100% charge degrades cathodes faster.
- Deep Discharges: Draining to 0% strains anode materials.
How to Minimize Damage (Step-by-Step)
- Avoid Fast Charging: >1C rates (e.g., 0% to 80% in 30 mins) increase heat.
- Partial Cycles: Discharging to 20% and charging to 80% doubles cycle life vs. 0–100%.
- Temperature Control: Store batteries at 15–25°C (59–77°F).
Electrochemical Reactions and Gradual Degradation
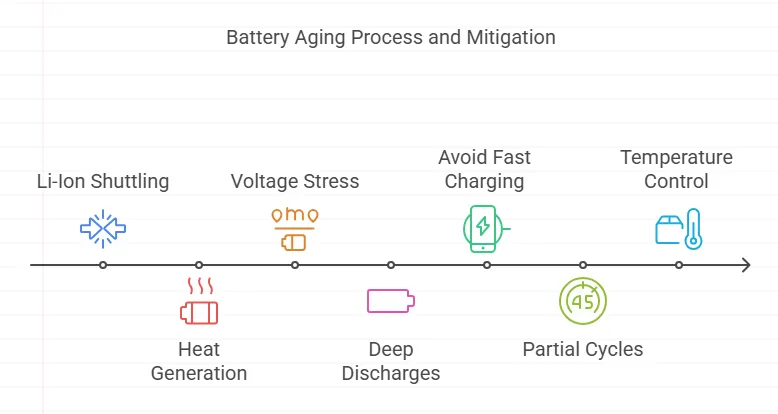
Why Do Batteries Degrade Over Time?
- SEI Layer Growth: A protective film on the anode thickens, blocking lithium ions.
- Metal Dissolution: Cobalt/Nickel from cathodes contaminates electrolytes.
- Mechanical Cracking: Repeated ion insertion expands anode materials (e.g., graphite).
How Chemistry Affects Degradation Rates
- High-Voltage Cells (>4.2V): Faster electrolyte oxidation.
- Low-Temperature Operation: Causes lithium plating (metallic deposits that short-circuit cells).
Industrial/Grid Storage Battery Longevity Challenges
Why Large-Scale Batteries Age Differently
- Deep Cycling: Grid batteries often discharge fully, unlike consumer electronics.
- Scaled-Up Stress: A 1MWh system faces uneven cell aging, complicating maintenance.
How Operators Extend Lifespan
- Active Balancing: Electronics redistribute charge among cells.
- Predictive Algorithms: Monitor voltage/thermal data to replace weak cells early.
How Temperature Affects Lithium-Ion Battery Degradation
What Happens to Batteries in Extreme Temperatures?
Lithium-ion batteries degrade faster in both high heat and extreme cold due to accelerated chemical reactions:
-
High Temperatures (>40°C/104°F):
- Electrolyte breaks down, forming gas and increasing internal resistance.
- Cathode materials oxidize, reducing capacity.
-
Low Temperatures (<0°C/32°F):
- Lithium ions plate onto the anode instead of intercalating, causing permanent capacity loss.
- Electrolyte viscosity increases, slowing ion movement.
Why Does Temperature Matter for Longevity?
- A battery stored at 25°C (77°F) retains ~80% capacity after 1,000 cycles, but at 40°C (104°F), it may drop to 60% in half the cycles.
- Cold temperatures temporarily reduce performance (e.g., slower EV charging in winter).
How to Minimize Temperature Damage (Step-by-Step)
- Avoid Direct Sunlight/Heat Sources: Store devices away from radiators or hot cars.
- Use Thermal Management Systems: EVs and grid batteries use liquid cooling/heating.
- Pre-Warm Batteries in Cold Climates: Some EVs precondition batteries before fast charging.
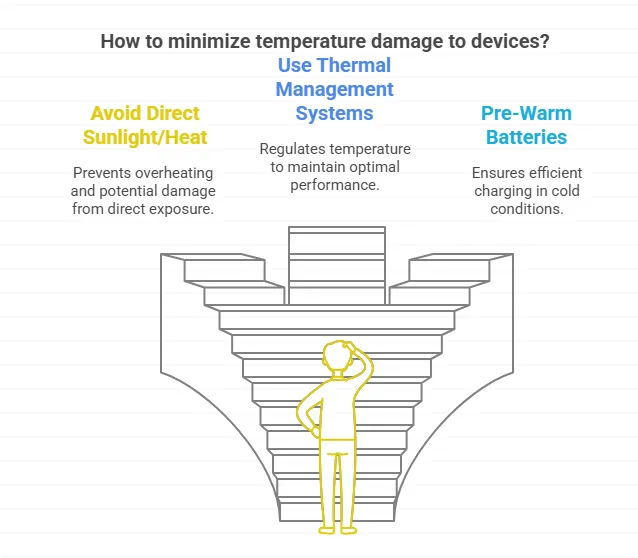
Cycle Testing vs. Calendar Life Testing: What’s the Difference?
What Are These Tests?
- Cycle Testing: Measures battery life by repeating charge/discharge cycles (e.g., 0%–100%) until capacity degrades to 80%.
- Calendar Life Testing: Evaluates aging by storing batteries at a fixed charge (e.g., 50%) and temperature without cycling.
Why Are Both Tests Important?
- Cycle Testing predicts lifespan for frequently used devices (phones, EVs).
- Calendar Testing matters for backup storage (grid batteries, rarely used devices).
How Testing Works (Step-by-Step)
-
Cycle Testing:
- Charge to 100%, discharge to 0%, repeat.
- Track capacity loss every 100 cycles.
-
Calendar Testing:
- Store batteries at 50% charge and elevated temperatures (e.g., 40°C) to simulate years of aging in months.
Avoiding Overcharging and Deep Discharging
Why Are These Practices Harmful?
-
Overcharging (>100%):
- Forces excess lithium into the anode, causing cracks.
- Oxidizes the electrolyte, generating heat and gas.
-
Deep Discharging (<0%):
- Copper anode current collectors dissolve, leading to short circuits.
How to Protect Your Battery (Step-by-Step)
- Use Smart Chargers: They stop charging at 100% or switch to trickle charging.
- Avoid Draining to 0%: Most devices shut down before true 0%, but frequent deep discharges hurt longevity.
- Set Charge Limits: Some EVs/phones allow capping at 80–90% for daily use.
How to Store Batteries Long-Term Without Damage
What Causes Battery Damage During Storage?
- High Charge Levels (100%): Accelerates electrolyte breakdown.
- Low Charge Levels (0%): Risks deep discharge and copper dissolution.
- Temperature Fluctuations: Heat/cold speeds up aging.
How to Store Batteries Properly (Step-by-Step)
- Charge to 40–60%: Ideal for minimizing stress on electrodes.
- Store in a Cool, Dry Place: 15–25°C (59–77°F) is optimal.
- Check Every 6 Months: Recharge to 40–60% if voltage drops below 3.0V/cell.
This article delves into the complexities of lithium-ion battery life, starting with the fundamental metrics of cycle life and capacity retention. It examines how cell design choices, including anode materials and cathode chemistries, influence battery longevity. The article also highlights the impact of charging and discharging practices on battery aging and offers practical advice to minimize damage. Additionally, it explores the challenges of managing large-scale industrial and grid storage batteries and the role of temperature in battery degradation. By understanding these factors, readers can take proactive steps to maximize the lifespan of their lithium-ion batteries.